Principles and safe use of electrosurgery in minimally invasive surgery
Introduction
Electrosurgery is the most commonly used form of surgical energy due to its availability, low cost and versatility. In spite of its wide use, there is evidence that surgeons of different grades and specialties have gaps in their knowledge of electrosurgery and this can compromise patient safety (1,2). The goal of this review is to bridge such gaps by addressing the principles, hazards and safe use of electrosurgery.
Historical overview
Thermal cautery was the main energy tool used by early surgeons for thousands of years. Although it was known in various ancient cultures, its earliest documented use was found in the Edwin Smith papyrus, dated to around 3000 BC. It is the oldest medical text that presents a rational and scientific approach to medicine in ancient Egypt. The history of electrosurgery was closely linked to the history of electricity (3). In the early 19th century, Becquerel was the first to use electrocautery. He used a direct electric current to heat a wire to achieve haemostasis without any electricity passing through the patient. The year 1881 was an important milestone in the development of electrosurgery. It was then that Morton described a safe alternating current with a frequency of 100 KHz which did not cause neuromuscular stimulation or electric shock when applied to the human body. Ten years later, d’Arsonval demonstrated that such high-frequency alternating current caused increased tissue temperature. Later, Nagelschmidt utilized that heating effect to treat joint and vascular diseases and called it diathermy in 1897. Rivere, one of d’Arsonval’s students, was credited as the first to use electrosurgery to treat a hand ulcer in 1900. The above developments among others paved the way for Bovie to build his electrosurgical generator that was later used by Cushing in his neurosurgical cases in 1926. It led to dramatic reductions in the perioperative morbidity and mortality of such cases (4). Bovie’s generator promoted the use of electrosurgery and ushered its modern era.
Principles of electrosurgery
Electrosurgery is the surgical application of high-frequency electricity to cause the thermal tissue effects of vaporisation, desiccation, coagulation and fulguration. It is not synonymous with electrocautery which is cautery using an electrically heated instrument with no electricity passing through the cauterised tissue. Electric current can be direct when it flows in one direction or alternating when it changes its direction regularly (Figure 1). Electrosurgery uses alternating current whereas electrocautery uses direct current. Electrosurgery follows the basic rules of electricity. Current is simply the flow of electrons pushed by the voltage through the impedance (resistance) of the circuit. In Ohm’s law, current in amperes (Amp) is equal to the voltage in volts divided by the resistance in ohms [Current (I) = Voltage (V)/Resistance (R)]. Consequently, to increase the current, you have to increase the voltage or reduce the resistance. Power is the amount of energy produced (or consumed) per unit time and is measured in watts. Power (P) = Voltage (V) × Current (I) or P = V2/R. So, in practice, when resistance increases, voltage will also increase to maintain the power needed to produce the desired tissue effect. Interestingly, high voltage is associated with increased electrosurgical complications. The electrosurgical circuit is dipole consisting of a generator, two electrodes and the patient. Dipole circuits can be monopolar or bipolar. In a monopolar circuit, the narrow active electrode concentrates the electric current on the target tissue whereas the wide dispersive electrode is attached remotely on the patient to dissipate the current safely. On the other hand, in a bipolar circuit the two equal electrodes are located at the target tissue with the electric current confined to the tissue between the two electrodes (Figure 2) (5).
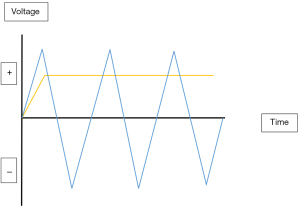
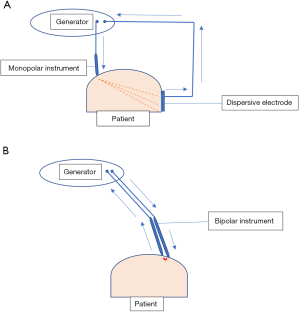
How electrosurgery works
During electrosurgery, the back and forth movements of the high-frequency alternating current make the cellular ions oscillate to create frictional heat. Thus, electrical energy is converted to mechanical then to thermal energy intracellularly. Such generated heat produces the thermal tissue effects. Alternating electrical currents with frequencies higher than 100,000 Hz are not associated with the Faradic effect of neuromuscular stimulation when applied to patients. In addition, the use of such high-frequency alternating currents would lead to more predictable and controllable electrosurgical tissue effects.
Electrosurgical units (generators) change the low-frequency of household electricity (50–60 Hz) to higher frequencies of 500,000–3,000,000 Hz. In addition, they control the wattage, voltage and duty cycle of the current. Generally, they produce three fundamental current waveforms; cut, blend and coagulation (coag) (Figure 3). Modern smart generators have additional functions such as monitoring tissue impedance to adjust their power output for uniform tissue effects.
Thermal tissue effects
Figure 4 shows the temperature levels at which different tissue effects take place.
Vaporisation
Electrosurgical tissue cutting occurs when temperature rises rapidly to 100 °C or more leading to cellular vaporization and explosion. With pure cut (continuous waveform through a pointed electrode with no tissue contact), there is minimal thermal damage at the edges of the incision as heat is dissipated into the resulting steam envelope. Although the cut (continuous) waveform is commonly used in cutting tissue, the blend and coag (interrupted) waveforms can also be used in cutting by increasing the power output or decreasing the active electrode size to achieve high current density. The latter cutting is associated with more lateral thermal damage due to the associated high voltage.
Desiccation and coagulation
Gradual rise of temperature between 60–95 °C will produce desiccation and coagulation instantaneously. Desiccation occurs due to the loss of cellular water through affected cell membrane. On the other hand, coagulation occurs due to thermal protein denaturation. The three basic waveforms can cause desiccation and coagulation when current density is low due to the use of a wider active electrode in the contact mode.
Fulguration
Fulguration occurs when the coag waveform is used with the active electrode not in contact with the tissue. The high voltage produces electric arcing to bridge the air gap between the active electrode and the tissue. This arcing creates a high temperature of 200 °C or more and causes carbonisation. As the duty cycle is low, the current diminishes rapidly and creates minimal heat in the deeper layers hence the thin carbonization layer. Fulguration is effective in controlling blood ooze from a raw surface area.
Variables that modify tissue effects
Generator mode (current waveform)
Generators have three basic modes which can be used to produce different tissue effects. The cut mode produces a continuous sine waveform with low voltage, high current and 100% duty cycle. Such mode is yellow-coded. The coag mode produces an interrupted waveform with high voltage, low current and 6% duty cycle. It is blue-coded. The tissue effects of the above waveforms are modified by the size and shape of the active electrode and whether there is contact or noncontact with the tissues. The third mode is the ‘blend’ which is a modulated cut mode with a modulated waveform of variable voltage, current and duty cycle. It is yellow-coded. Such waveform produces a combination of tissue cutting with desiccation and coagulation, so it is used when haemostasis is required with cutting. Some generators have blend 1, 2 and 3 with duty cycle at 50%, 40% and 25% respectively (Figure 3). The higher its duty cycle the more cutting and less coagulation it produces and vice versa. As each of the three modes can produce both cutting and coagulation, the terms cut and coag modes are misleading. The three modes are better described as continuous low-voltage, interrupted high-voltage and interrupted low-voltage respectively (6).
Power setting
As power output is directly related to electrosurgical complications, surgeons are advised to use the lowest effective power output. In the cut mode, 50–80 W are generally used for effective cutting whereas 30–50 W produce effective coagulation in the coag mode (7).
Active electrode shape (current density)
Simply, electrosurgery operates by focusing the electric current at the active electrode to achieve the required tissue effects and by defocusing the current at the dispersive (return) electrode when used to prevent unwanted burns. The cutting active electrode is usually pointed (needle or hook) to produce high current density. In contrast, the coagulating active electrode is broad to produce low current density. In fulguration, the active electrode is usually spherical to spray coagulation on a wide area.
Dwell time
Prolonged contact between the active electrode and tissue results in wider and deeper tissue effects and unintended thermal injuries, whereas too short a dwell time produces inadequate tissue effects. In the cut mode, the ideal cutting speed produces a clean cut with minimal lateral thermal spread. Moving the electrode too slowly will create a zone of desiccation and coagulation along the cut. If the electrode is moved too fast ahead of the steam envelope, contact of the electrode with the tissue results in desiccation and coagulation as current density decreases.
Electrode-tissue interface
In the cut mode, arcing and linear vaporisation occur as the pointed active electrode is advanced very close to the tissue (near-contact). Alternatively, if such electrode touches the tissue, coagulation happens as current density is reduced at the point of contact. As previously stated, fulguration is achieved through non-contact as the active electrode sprays superficial coagulation on a large surface area. Desiccation and coagulation occur when a flat active electrode touches the tissue. A monopolar or bipolar grasping forceps can achieve coaptive coagulation when compressing the tissue between their jaws during activation.
Tissue factors
Tissues with high impedance such as fat and scar tissue will require higher power to achieve the desired tissue effects compared to those with low impedance such as muscles and skin. Generally, obese or emaciated patients need more power output to cause the same tissue effects as in lean and muscular patients. Patients with vascular diseases such as atherosclerosis, liver cirrhosis, diabetes and collagen disorders may not be suitable for electrosurgical haemostasis and alternative haemostatic techniques may be required.
Eschar
Eschar build-up on the active electrode poses a high impedance to current hence higher power will be needed to achieve the desired tissue effects. It is good practice to keep the electrode clean at all times or to use non-stick electrodes (Teflon or Silicone coated).
Electrosurgical technique
The art of using electrosurgery to produce the intended effects without the associated risks, depends on the masterful knowledge of electrosurgical instruments and the skilful application of the above factors to produce the desired tissue effects with the lowest effective power output.
Monopolar instruments
They are versatile and can produce several tissue effects such as pure cutting, cutting with haemostasis, coagulation, fulguration and coaptive coagulation of small blood vessels.
Traditional bipolar instruments
The early use of laparoscopic monopolar instruments was associated with serious hazards which led to the development of the traditional bipolar instruments to overcome such hazards. In a bipolar electrosurgical circuit, there is no dispersive electrode and the current flows only in the tissue between the two jaws of the bipolar instrument hence low voltage with 100% duty cycle (cut waveform) is used (Figure 3). In spite of being generally safer than their monopolar counterparts, the traditional bipolar instruments have their own limitations. They use uninterrupted energy delivery and rely on visual clues (vapour and colour changes) to assess adequacy of coagulation. Also tissue coaptation is not always good with often incomplete coagulation and haemostasis.
Advanced bipolar vessel-sealing devices
Such devices were developed to address the above limitations and they have actually revolutionised modern laparoscopic surgery with increased uptake of more complex procedures. Their jaws compress, coagulate and seal blood vessels of up to 7 mm. They also transect the sealed tissues using the bipolar energy or other built-in mechanisms. These devices have tissue feedback mechanisms to adjust the power output for adequate and consistent tissue effects. They use less energy compared to the traditional bipolar devices with less thermal spread and smoke production. Their output is pulsed to give time for the tissues to cool down during the off phase (8). The number of such devices is increasing on the market with scarce studies to assess them (9-12). Most of these studies were laboratory or animal based and showed that such devices produced a seal with supraphysiological burst pressure (>250 mmHg) (13). The small variations of such high pressure among various devices have no bearing on surgical outcomes. Such outcomes were assessed in terms of operation time, blood loss, complications, postoperative pain and length of hospital stay (14). In addition, the cost-effectiveness of these devices was assessed in some studies (15,16). Surgeon’s preference is often the determining factor in the choice of the energy device used. There is a need for more clinical studies to compare the various surgical outcomes of these devices so as to help surgeons choose the appropriate device for their procedure.
Hazards of electrosurgery
Although advanced technology has significantly reduced electrosurgical complications, serious internal burns still occur. The estimated incidence of such burns is 3.6 per 1,000 laparoscopic procedures. The majority of such burns are not recognised at the time of surgery, which can lead to severe morbidity or even mortality postoperatively. In addition, they are associated with increased cost due to repeated surgery, prolonged hospitalization and malpractice claims.
Table 1 shows a practical classification of electrosurgical hazards.
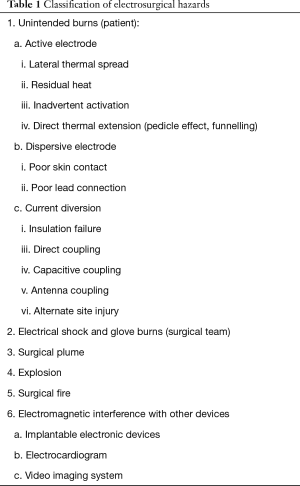
Full table
Lateral thermal spread
Lateral thermal spread is the most common cause of electrosurgical unintended burns with serious sequalae when the bowel, ureter and blood vessels are affected (17). The extent of such injury is determined by the device used, power output, dwell time and tissue impedance. Monopolar devices generate more lateral thermal spread during coagulation compared to the bipolar and ultrasonic (18).
Residual heat
Different energy devices retain heat after deactivation for a variable length of time. Ultrasonic devices have the highest residual heat compared to the monopolar, bipolar and argon beam coagulator (19). Surgeons should avoid touching important structures with their energy devices before they cool down after deactivation.
Inadvertent activation
Inadvertent activation of electrosurgical devices may result in unintended burns so such devices should be kept alone in a dry plastic holster when not in use (5).
Direct thermal extension (pedicle effect, funnelling)
It occurs when the active electrode touches a structure with a narrow pedicle or a narrow band of adhesion (Figure 5). The increased current density at that remote narrow area causes an unintended burn (20). To reduce such a risk, use the lowest effective power with short activation time (2–3 seconds) or preferably avoid monopolar devices in these areas.
Dispersive electrode burns
The design of the dispersive electrode with a wide surface area creates low current density to dissipate the current safely. It is to be well applied to a muscular area with low impedance. It should be close to the operation site but away from any metal prosthesis. Skin areas with increased impedance such as irregular body contour, bonny prominences, fat, scar tissue and hairy skin should be avoided. Dispersive electrode burns occur when there is inadequate electrode contact with the patient due to either partial detachment of the dispersive electrode or high impedance at the skin application area. The associated high current density results in such burns. Electrosurgical burns show up at the conclusion of surgery but burns showing up later are not electrosurgical. Contact quality monitoring (CQM) technology was developed in 1980s to prevent such burns. It uses a split pad with a generator processor monitoring the amount of contact as well as the impedance of the applied split pad. In case of poor contact or high impedance, the system will alarm and stop the generator (21).
Insulation failure
It is a defect in the insulation layer of the active electrode. It is like having an extra active tip and can transmit the full wattage to nearby tissues. About 20% of reusable and 3% of single-use laparoscopic instruments have such defects mostly in their distal third (22). Robotic instruments have more insulation failure than the laparoscopic ones (23). It is important to note that the insulation defect can develop during the surgery when high voltage is used. The majority of these defects are too small to be detected by inspection and lead to high current density and severe burns (24). Special detectors are used to identify these defects before surgery. Active electrode monitoring (AEM) technology was introduced in 1990s to tackle both insulation failure and capacitive coupling (25). It is formed of AEM instruments and the AEM monitor which can be fitted to most generators. Compared to traditional instruments, the AEM instrument has two extra layers: conductive shield and outer insulation (Figure 6). The conductive shield acts as a second dispersive electrode to return stray currents to the AEM monitor and to stop the ESU when such currents are high (Figure 7).
Direct coupling
It occurs when the activated electrode touches another metal instrument that may be in contact with some tissue as bowel. Such tissue can sustain thermal injury as a result. It is technique related and surgeons should keep the tip of the active electrode in view before activation. Another situation of direct coupling can occur if the active electrode is used to buzz a bleeding point along a staple line, which can generate metal-to-metal arcing of 1,000 °C and melting of staples. Never do that!
Capacitive coupling
A capacitor is formed when the active electrode induces current through its intact insulation in a nearby conductor. The induced current in the second conductor can cause serious burns. The magnitude of such induced current increases with the proximity of the two conductors, thinner insulation, increased voltage, and longer activation time (26). Also, longer instruments and narrower cannulas increase such risk (27). The use of plastic anchor (hybrid trocar) was abandoned as it prevented the dissipation of the induced current into the abdominal wall. Such trapped current in the metal cannula can pass to any nearby tissue causing unintended burns (28). The adaptive technology within modern generators can reduce that risk by delivering the lowest effective energy according to tissue feedback. In addition, AEM technology is designed to eliminate the risks from insulation failure and capacitive coupling.
Antenna coupling
This newly studied phenomenon in electrosurgery involves the emission of electromagnetic energy from the active electrode cord (emitting antenna) to be captured by a nearby inactive cord (receiving antenna). ECG wires, neuromonitors and camera cord can function as receiving antenna (29,30). It is similar to capacitive coupling and can lead to patient burns. To prevent such burns, it is recommended to separate the laparoscopy tower from the generator, avoid parallel arrangement of cords (Figure 8) and use lower wattage (31).
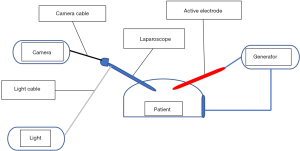
Alternate site burns
With the early ground-referenced generators, current passed from the generator to the active electrode, to the target tissue, to the dispersive electrode, back to the generator and finally to the ground. If the dispersive electrode was defective with high impedance, the current would exit the body at contact areas with metal to the ground. Current density would be high at such contact areas leading to alternate site burns. Such burns were common in those days but with the development of isolated generators, they nearly became a thing of the past.
Electrical shock and glove burns
Surgical gloves are used primarily to protect patients and surgical team from infection. Initially pre-existing holes in surgical gloves were thought to be responsible for the occasional shocks and burns that surgeons and assistants may receive through their gloves during electrosurgery. There are in fact three mechanisms where leaked current can pass through the gloves; direct current conduction, capacitive coupling and high-voltage dielectric breakdown. To minimise this risk, one should use the lowest effective power output, avoid open and prolonged activation as well as re-glove or double glove for prolonged surgery.
Surgical smoke
A hazardous gaseous by-product is formed during surgery when energy devices are used. It is a common occurrence due to the wide use of surgical energy devices. It is formed of 95% water vapour that acts as a carrier for the 5% other materials. Smoke with high levels of small particles reduces laparoscopic visibility which can lead to complications. Bipolar and ultrasonic devices were reported to produce less smoke with less affected visibility compared to the monopolar (32). Surgical smoke can cause irritation of the eyes and upper respiratory tract of theatre staff. Small particles <5 µ can reach the bronchioles and alveoli and potentially cause bronchiolitis, emphysema and pulmonary fibrosis. As with cigarette smoke, surgical smoke contains toxic and mutagenic substances. Whether long-term exposure to surgical smoke causes cancer in humans is not certain with a study showing that the risk of lung cancer is not increased in theatre nurses (33). In addition to the harmful chemicals, surgical smoke may contain viable malignant cells, live bacteria and viruses which can put attending staff at risk of infection. Hepatitis B virus was isolated from surgical smoke during laparoscopic surgery (34). In addition, there are case reports of HPV positive tonsillar cancer in two gynaecologists who had years of exposure to surgical smoke resulting from the treatment of HPV-related cervical and vulvar lesions (35). The strategy to reduce such potential hazards is three-stepped: reduction of smoke production, safe smoke evacuation and the use of adequate personal protective equipment (PPE). Safe and masterful use of electrosurgery is associated with less smoke compared to tissue charring.
In the current covid-19 pandemic, there is a justified concern about the potential risk of viral transmission through aerosolisation and surgical smoke. Various scientific societies published recommendations of varying level of evidence to reduce that possible risk. Such recommendations use the above strategy with emphasis on the use of closed-circuit smoke evacuation devices attached to ultra-low particulate air (ULPA) filters which can filter 99.95% of particles 30.12 µ. All operating room staff should wear the appropriate PPE such as FFP3 respirators that filter particles >0.3 µ (36).
Explosions
Although surgical explosions are rare, they can be potentially fatal. Flammable anaesthetics such as ether and cyclopropane, are no longer used in most countries as they were associated with significant risk of explosions and fires. Like fires, explosions require three factors to occur: oxygen, fuel and spark (Figure 9). There were early reports of explosions during colonoscopic use of electrosurgery due to the accumulation of the flammable bowel gasses: hydrogen and methane (37). Bowel preparation is recommended before the procedure to reduce such risk. Also, bladder explosion and rupture have been reported during transurethral electrosurgical resection of the prostate and bladder polyps where hydrogen was produced during the resection (38). During laparoscopy, such explosions were reported with electrosurgery when nitrous oxide, oxygen or a mixture of oxygen and carbon dioxide were used to establish pneumoperitoneum. In addition, bowel perforation can leak hydrogen and methane into the peritoneal cavity thus creating an explosion risk (38). To prevent such rare explosions, pure carbon dioxide is to be used for pneumoperitoneum.
Surgical fires
Although operating room fires are never events, they still occur and can potentially cause devastating outcomes (39). In addition, their medico-legal costs are high (40). Such surgical fires occur when the three elements of fire triangle: oxidizer, heat and fuel co-exist (Figure 9). More than 81% of such fires involve surgical drapes whose flammability increases as oxygen concentration rises (41). The high concentration of supplemental oxygen is the most important factor of fire triangle with most fires occurring when its concentration exceeds 30%. The FDA recommends routine fire risk assessment before surgery. Such assessment can be an integral part of the WHO preoperative timeout. Such assessment highlights the importance of effective communication among all members of surgical team to prevent surgical fires. The following are broad recommendations to prevent surgical fires:
- Oxidiser
- Use a closed rather than an open oxygen system when appropriate;
- Avoid oxygen accumulation underneath tented drapes.
- Ignition source
- Avoid energy devices in surgery of the head, neck and chest when high concentrations of oxygen are used;
- Do not use energy devices to enter the trachea during tracheostomy;
- When not in use, place electrosurgical devices in holster away from patient and drapes.
- Fuel
- Avoid alcoholic skin preps if possible;
- Allow enough time (more than 3 minutes) for alcoholic skin preps to dry;
- Avoid pools of alcoholic skin preps (42).
Although rapid recognition of surgical fire is paramount, alcohol-based prep fires present a challenge due to their faint blue flames that are masked by blue surgical drapes. To control a surgical fire, several tasks need to be undertaken almost simultaneously by all theatre team:
- Stop airway gases to get rid of the high oxygen environment. In case of airway fires remove the endotracheal tube and pour saline in the airway;
- Use water, saline and wet towels to stop the fire;
- Remove burning materials from patient;
- Carbon dioxide extinguisher can be used to control the fire on the burning material;
- Patient is cared for in a safe area where intubation is re-established to restore breathing with room air.
Electromagnetic interference (EMI) with other devices
Implantable electronic devices
The use of implantable electronic devices such as pacemakers, cardioverter-defibrillators and neuro-stimulators is on the increase and hence operating on patients with such devices is not uncommon. The electromagnetic fields associated with electrosurgery can interfere with the function of such devices, which can lead to adverse effects such as hypotension, myocardial injury arrhythmias and asystole (43,44). The clinical effect depends to a large extent on patient dependency on the implanted device. The early reported hazards of electrosurgery in pacemaker patients, have led to the advancement of pacemakers and the development of guidelines for safe use of electrosurgery in such patients. To prevent such serious problems, the approach should be patient-specific and multidisciplinary with good communication among the cardiologist, surgeon, anaesthetist and theatre staff (45,46). The use of monopolar devices in procedures below the umbilicus is unlikely to interfere with pacemakers and cardioverter-defibrillators. It is important to liaise with the cardiologist before the surgery to assess patient’s dependence on the device, evaluate the device function and to have a management plan. In case of pacemakers, it is better to programme the device to asynchronous mode and deactivate rate adaptive pacing and the tachy therapy of cardioverter-defibrillators.
During surgery, it is advisable to use bipolar or ultrasonic instruments especially in device-dependent patients. If monopolar devices have to be used:
- Place dispersive electrode to keep current path away from implanted device;
- Place the generator away from the implanted device;
- Use short bursts at the lowest effective energy;
- Use the cut rather than the coag mode (less voltage);
- Do not move the activated electrode over the implanted device.
As EMI can affect electrocardiogram (ECG), an additional method is to be used to check the peripheral pulse (pulse oximetry, arterial line or palpation) with availability of temporary pacing and defibrillation equipment. After surgery, patient remains in a monitored environment with accessible equipment for external pacing and cardio-version-defibrillation, till the preoperative settings of the device are restored.
ECG
In addition to the alternate site burns at the ECG pads, electrosurgery can cause distortion of the ECG signal. Much of this distortion is caused by electrosurgical arcing. To reduce such interference:
- Increase the distance between the leads of the generator and ECG;
- Use the lowest effective power;
- In cut mode, use short activations to decrease the effect of arcing;
- Avoid open activation.
Video imaging system
The flow of high frequency electric current within the electrosurgical circuit is associated with electromagnetic waves in the space surrounding the circuit wires. As the electrosurgical frequencies are similar to those of the video system, such waves can interfere with the video imaging system of laparoscopy. The interference on the video screen can range from noise spots. This interference is increased with higher power output, higher voltage, electrosurgical arcing and the close proximity of the electrosurgical generator to the video system. Modern electrosurgical units have automatic power control circuit, which adjusts the power output to the lowest effective wattage. This reduces the above interference. In addition, most modern video systems have electromagnetic shielding to reduce the interference. The simplest way of reducing such interference is to separate the video system from the electrosurgical unit.
Training in electrosurgery
Electrosurgery is used by most surgeons, sometimes without robust training in its principles and its safe use. This can compromise patient safety. Several studies showed that most surgeons poorly understand electrosurgery (1,2,47,48). The Society of American Gastrointestinal and Endoscopic Surgeons (SAGES) took the initiative and developed its validated training program in electrosurgery which is knowledge-based (5). Interestingly, Madani et al. (49) showed that adding a practical part to the programme enhanced the learning outcomes of the participants.
Conclusions
Electrosurgery is a very useful surgical tool. Improvements in technology increased its safety and reduced complications. It facilitates surgery as a versatile tool but training is essential to understand its principles and reduce complications.
Acknowledgments
Funding: None.
Footnote
Provenance and Peer Review: This article was commissioned by the Guest Editor (Gokhan Kilic) for the series “Minimally Invasive Treatment Modalities for Female Pelvic Floor Disorders” published in Gynecology and Pelvic Medicine. The article has undergone external peer review.
Conflicts of Interest: Both authors have completed the ICMJE uniform disclosure form (available at https://gpm.amegroups.org/article/view/10.21037/gpm-2020-pfd-10/coif). The series “Minimally Invasive Treatment Modalities for Female Pelvic Floor Disorders” was commissioned by the editorial office without any funding or sponsorship. ES reports personal fees from Olympus UK, personal fees from Johnson and Johnson, outside the submitted work. Both authors have no other conflicts of interest to declare.
Ethical Statement: The authors are accountable for all aspects of the work in ensuring that questions related to the accuracy or integrity of any part of the work are appropriately investigated and resolved.
Open Access Statement: This is an Open Access article distributed in accordance with the Creative Commons Attribution-NonCommercial-NoDerivs 4.0 International License (CC BY-NC-ND 4.0), which permits the non-commercial replication and distribution of the article with the strict proviso that no changes or edits are made and the original work is properly cited (including links to both the formal publication through the relevant DOI and the license). See: https://creativecommons.org/licenses/by-nc-nd/4.0/.
References
- Mayooran Z, Pearce S, Tsaltas J, et al. Ignorance of electrosurgery among obstetricians and gynaecologists. BJOG 2004;111:1413-8. [Crossref] [PubMed]
- Meeuwsen F, Guédon A, Klein J, et al. Electrosurgery: short-circuit between education and practice. Minim Invasive Ther Allied Technol 2019;28:247-53. [Crossref] [PubMed]
- Sutton C, Abbott J. History of power sources in endoscopic surgery. J Minim Invasive Gynecol 2013;20:271-8. [Crossref] [PubMed]
- Massarweh N N, Cosgriff N, Slakey D P. Electrosurgery: History, principles, and current and future uses. J Am Coll Surg 2006;202:520-30. [Crossref] [PubMed]
- El-Sayed M, Mohamed S, Saridogan E. Safe use of electrosurgery in gynaecological laparoscopic surgery. The Obstetrician & Gynaecologist 2020;22:9-20. [Crossref]
- Law KSK, Abbott JA, Lyons SD. Energy Sources for Gynecologic laparoscopic surgery: A review of the literature. Obstet Gynecol Surv 2014;69:763-76. [Crossref] [PubMed]
- Wang K, Advincula AP. “Current thoughts” in electrosurgery. Int J Gynaecol Obstet 2007;97:245-50. [Crossref] [PubMed]
- Davison J, Zamah N. Electrosurgery: Principles, biologic effects, and results in female reproductive surgery. Glob Libr Women's Med (ISSN: 1756-2228) 2008. DOI: 10.3843/GLOWM.10021. [Crossref]
- Person B, Vivas DA, Ruiz D, et al. Comparison of four energy-based vascular sealing and cutting instruments: A porcine model. Surg Endosc 2008;22:534-8. [Crossref] [PubMed]
- Newcomb WL, Hope WW, Schmelzer TM, et al. Comparison of blood vessel sealing among new electrosurgical and ultrasonic devices. Surg Endosc 2009;23:90-6. [Crossref] [PubMed]
- Klar M, Haberstroh J, Timme S, et al. Comparison of a reusable with a disposable vessl-sealing device in a sheep model: efficacy and costs. Fertil Steril 2011;95:795-8. [Crossref] [PubMed]
- Entezari K, Hoffmann P, Goris M, et al. A review of currently available vessel-sealing systems. Minim Invasive Ther Allied Technol 2007;16:52-7. [Crossref] [PubMed]
- Okhunov Z, Yoon R, Lusch A, et al. Evaluation and comparison of contemporary energy-based surgical vessel-sealing devices. J Endourol 2018;32:329-37. [Crossref] [PubMed]
- Jaiswal A, Huang KG. Energy devices in gynaecological laparoscopy - Archaic to modern era. Gynecol Minim Invasive Ther 2017;6:147-51. [Crossref] [PubMed]
- Munro MG. Economics and energy sources. J Minim Invasive Gynecol 2013;20:319-27. [Crossref] [PubMed]
- Holloran-Schwartz MB, Gavard JA, Martin JC, et al. Single-use energy sources and operating room time for laparoscopic hysterectomy: a randomised controlled trial. J Minim Invasive Gynecol 2016;23:72-7. [Crossref] [PubMed]
- Overbey DM, Townsend NT, Chapman BC, et al. Surgical energy-based device injuries and fatalities reported to the Food and Drug Administration. J Am Coll Surg 2015;221:197-205.e1. [Crossref] [PubMed]
- Sutton PA, Awad S, Perkins AC, et al. Comparison of lateral thermal spread using monopolar and bipolar diathermy, the Harmonic Scalpel™ and the Ligasure™. Br J Surg 2010;97:428-33. [Crossref] [PubMed]
- Govekar HR, Robinson TN, Stiegmann GV, et al. Residual heat of laparoscopic energy devices: how long must the surgeon wait to touch additional tissue? Surg Endosc 2011;25:3499-502. [Crossref] [PubMed]
- Humes DJ, Ahmed I, Lobo DN. The Pedicle effect and direct coupling: delayed thermal injuries to the bile duct after laparoscopic cholecystectomy. Arch Surg 2010;145:96-8. [Crossref] [PubMed]
- Odell RC. Surgical complications specific to monopolar electrosurgical energy: engineering changes that have made electrosurgery safer. J Minim Invasive Gynecol 2013;20:288-98. [Crossref] [PubMed]
- Montero PN, Robinson TN, Weaver JS, et al. Insulation failure in laparoscopic instruments. Surg Endosc 2010;24:462-5. [Crossref] [PubMed]
- Espada M, Munoz R, Noble BN, et al. Insulation failure in robotic and laparoscopic instrumentation: a prospective evaluation. Am J Obstet Gynecol 2011;205:121.e1-5. [Crossref] [PubMed]
- Yazdani A, Krau H. Laparoscopic instrument insulation failure: the hidden hazard. J Minim Invasive Gynecol 2007;14:228-32. [Crossref] [PubMed]
- Vancaillie TG. Active electrode monitoring. How to prevent unintentional thermal injury associated with monopolar electrosurgery at laparoscopy. Surg Endosc 1998;12:1009-12. [Crossref] [PubMed]
- Vilos G, Latendresse K, Gan BS. Electrophysical properties of electrosurgery and capacitive induced current. Am J Surg 2001;182:222-5. [Crossref] [PubMed]
- Huang HY, Yen CF. WU MP. Complications of electrosurgery in laparoscopy. J Gynecol Minim Invasive Ther 2014;3:39-42. [Crossref]
- Wu MP, Ou CS, Chen SL, et al. Complications and recommended practices for electrosurgery in laparoscopy. Am J Surg 2000;179:67-73. [Crossref] [PubMed]
- Aigner N, Fialka C, Fritz A, et al. Complications in the use of diathermy. Burns 1997;23:256-64. [Crossref] [PubMed]
- Parikh SN, Mehlman CT, Keith RW. A third-degree burn caused by a neurogenic motor-evoked potential monitoring electrode during spinal surgery: a case report. Spine 2003;28:E21-4. [Crossref] [PubMed]
- Robinson TN, Barnes KS, Govekar HR, et al. Antenna coupling-a novel mechanism of radiofrequency electrosurgery complication: practical implications. Ann Surg 2012;256:213-8. [Crossref] [PubMed]
- Weld KJ, Dryer S, Ames CD, et al. Analysis of surgical smoke produced by various energy-based instruments and effect on laparoscopic visibility. J Endourol 2007;21:347-51. [Crossref] [PubMed]
- Gates MA, Feskanich D, Speizer FE, et al. Operating room nursing and lung cancer risk in a cohort of female registered nurses. Scand J Work Environ Health 2007;33:140-7. [Crossref] [PubMed]
- Kwak HD, Kim SH, Seo YS, et al. Detecting hepatitis B virus in surgical smoke emitted during laparoscopic surgery. Occup Environ Med 2016;73:857-63. [Crossref] [PubMed]
- Rioux M, Garland A, Webster D, et al. HPV positive tonsillar cancer in two laser surgeons: case reports. J Otolaryngol Head Neck Surg 2013;42:54. [Crossref] [PubMed]
- Vigneswaran Y, Prachand VN, Posner MC, et al. What Is the Appropriate Use of Laparoscopy over Open Procedures in the Current COVID-19 Climate? J Gastrointest Surg 2020;24:1686-91. [Crossref] [PubMed]
- Ladas SD, Karamanolis G, Ben-Soussan E. Colonic gas explosion during therapeutic colonoscopy with electrocautery. World J Gastroenterol 2007;13:5295-8. [Crossref] [PubMed]
- Macdonald AG. A brief historical review of non-anaesthetic causes of fires and explosions in the operating room. Br J Anaesth 1994;73:847-56. [Crossref] [PubMed]
- Choudhry AJ, Haddad NN. Surgical fires and operative burns: Lessons learned from a 33-year review of medical litigation. Am J Surg 2017;213:558-64. [Crossref] [PubMed]
- Mehta SP, Bhananker SM, Posner KL, et al. Operating room fires: a closed claims analysis. Anesthesiology 2013;118:1133-9. [Crossref] [PubMed]
- Culp WC, Kimbrough BA, Luna S. Flammability of surgical drapes and materials in varying concentrations of oxygen. Anesthesiology 2013;119:770-6. [Crossref] [PubMed]
- Jones EL, Overbey DM, Chapman BC, et al. Operating room fires and surgical skin preparation. J AM Coll Surg 2017;225:160-5. [Crossref] [PubMed]
- Meyer JP, Crew J. The risks of diathermy in the urological patient with a pacemaker or an automatic internal cardiac defibrillator. BJU Int 2008;101:528-9. [Crossref] [PubMed]
- Gunaruwan P, Barlow M. Diathermy-induced ventricular fibrillation with Riata high-voltage lead insulation failure. Europace 2013;15:473. [Crossref] [PubMed]
- The American Society of Anesthesiologists Committee on Standards and Practice Parameters. Practice advisory for the perioperative management of patients with cardiac implantable electronic devices: pacemakers and implantable cardioverter-defibrillators: an updated report by the American Society of Anesthesiologists task force on perioperative management of patients with cardiac implantable electronic devices. Anesthesiology 2011;114:247-61. [Crossref] [PubMed]
- Siddaiah-Subramanya M, Tiang KW, Nyandowe M. Complications, implications, and prevention of electrosurgical injuries: cornerstone of diathermy use for junior surgical trainees. Surg J 2017;3:148-53. [Crossref]
- Feldman LS, Fuchshuber P, Jones DB, et al. Surgeons don’t know what they don’t know about the safe use of energy in surgery. Surg Endosc 2012;26:2735-9. [Crossref] [PubMed]
- Hur HC, Green I, Modest AM, et al. Needs assessment for electrosurgery training of residents and faculty in obstetrics and gynecology. JSLS 2014;18:1-6. [Crossref] [PubMed]
- Madani A, Watanabe Y, Vassiliou MC, et al. Impact of a hands-on component on learning in the fundamental use of surgical energy (FUSE) curriculum: a randomized-controlled trial in surgical trainees. Surg Endosc 2014;28:2772-82. [Crossref] [PubMed]
Cite this article as: El-Sayed MM, Saridogan E. Principles and safe use of electrosurgery in minimally invasive surgery. Gynecol Pelvic Med 2021;4:6.